Research
The mechanism by which an earthquake occurs is very complicated. In our laboratory, we are conducting research to deepen our understanding of the seismic process based on seismic observations. We analyze seismic activity (foreshock activity) observed before major earthquakes that occurred in Japan and overseas, and analyze large-scale seismic waveform data acquired by a high-density, multipoint seismic observation network. For example, by improving the detection performance of seismic activity, we found that two slow slip transients occurred near the epicenter of the mainshock rupture before the 2011 Tohoku-Oki Earthquake (M9.0). Furthermore, it was clarified that a similar phenomenon occurred before the occurrence of the 2014 Northern Chile Earthquake (M8.2) and the 2016 Kumamoto Earthquake (M7.0). These foreshock activities are complex, with a wide variety of activities ranging from active to extremely low. I am working on research from the perspective of how such diversity arises and how slow slip transients are involved in the occurrence of earthquakes.
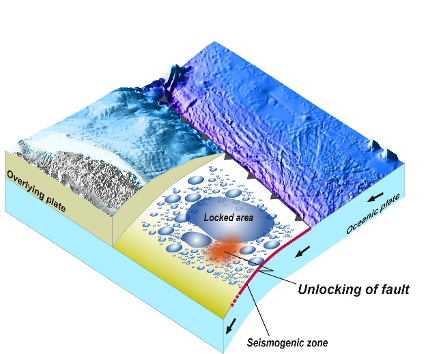
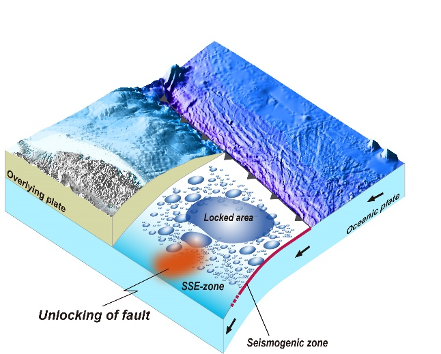
Fig. Schematic figures showing unlocking of plate boundary fault before large earthquakes. step- like increase in fault slip driven by a combination of migrating slow slip transients and foreshocks occurred before some megathrust earthquakes in subduction zones. The intermittent increase in fault slip loads nearby locked regions, increasing the likelihood of subsequent large earthquakes.
The generation of large earthquakes
Despite decades of observational, laboratory and theoretical studies, the processes leading to generation of large earthquakes remain enigmatic. However, recent observations provide new promising perspectives that advance the knowledge on the topic. Here, we review data on initiation processes of large earthquakes and show that they are multi-scale and diverse, involving localization of deformation, fault heterogeneities and variable local loading rate effects. Analyses of seismic and geodetic data reveal evidence for regional weakening by earthquake-induced rock damage and progressive localization of deformation around the eventual rupture zones a few years before some large earthquakes. The final phase of deformation localization includes (depending on conditions) a mixture of slow-slip transients and foreshocks at multiple spatial and temporal scales. The evolution of slip on large localized faults shows a step-like increase that might reflect stress loading by previous failures, which can produce larger dynamic slip, in contrast to the smooth acceleration expected for a growing aseismic nucleation phase. We propose an integrated model to explain the diversity of large earthquake generation from progressive volumetric deformation to localized slip, which motivates future near-fault seismic and geodetic studies with dense sensor networks and improved analysis techniques that can resolve multi-scale processes(Kato and Ben-Zion, 2021, Nature Reviews Earth & Environment, https://rdcu.be/caT5j).
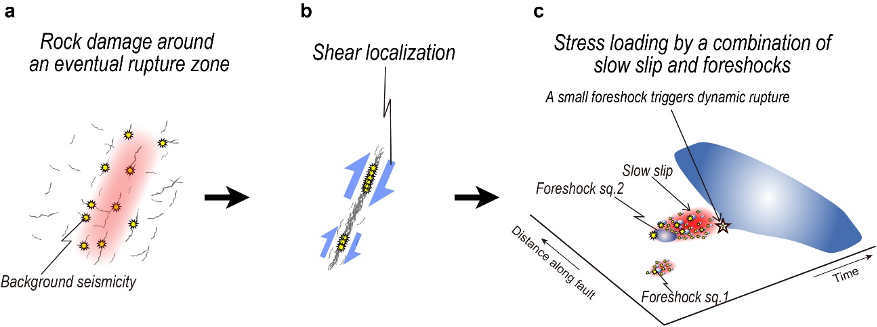
Fig. Schematic illustrations of generation processes of large earthquakes. (a) Progressive localization of shear deformation and background seismicity around a large rupture zone. (b) Shear localization and several foreshock sequences before the instability leading to the large rupture. (c) A space-time diagram of step-like increase in fault slip before a major earthquake, associated with combined slow slip and foreshocks. A final rapid local loading by a small foreshock triggers the subsequent major dynamic rupture and circumvents the large nucleation process of a large patch. White and yellow stars denote epicenters of mainshocks and other seismicity, respectively. As an example, two foreshock sequences accompanied with slow slip are displayed.
Conjugate faulting and structural complexity on the young fault system
Young faults display unique complexity associated with their evolution, but how this relates to earthquake occurrence is unclear. Unravelling the fine-scale complexity in these systems could lead to a greater understanding of ongoing strain localization in young fault zones. Here we present high-spatial-resolution images of seismic sources and structural properties along a young fault zone that hosted the Tottori earthquake (Mw 6.8) in southwest Japan in 2000, based on data from a hyperdense network of ~1,000 seismic stations. Our precise microearthquake catalog reveals conjugate faulting over multiple length scales. These conjugate faults are well developed in zones of low seismic velocity. A vertically dipping seismic cluster of about 200m length occurs within a width of about 10 m. Earthquake migrations in this cluster have a speed of about 30m per day, which suggests that fluid diffusion plays a role. We suggest that fine structural complexities influence the pattern of seismicity in a developing fault system (Kato et al., 2021, Communications Earth & Environment).
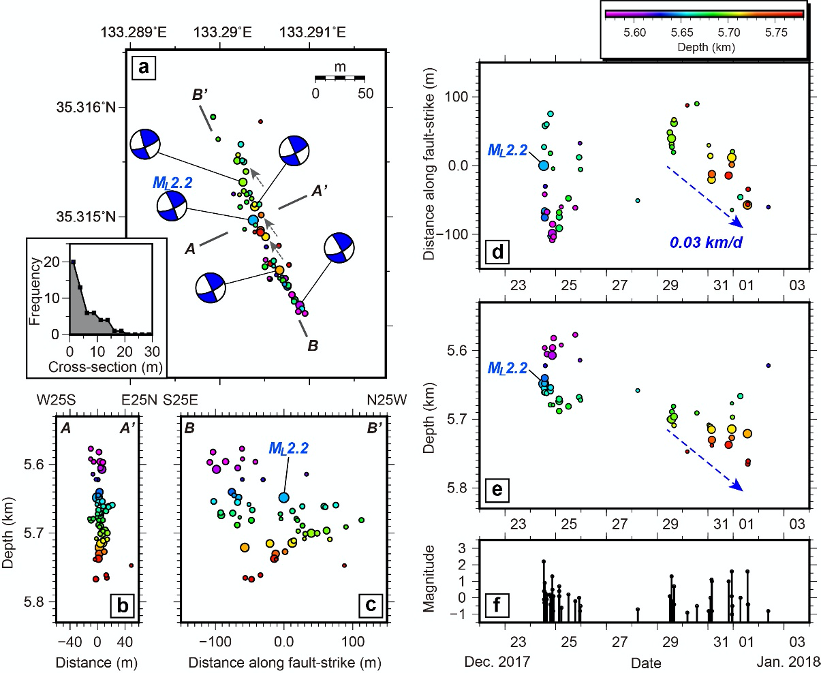
Fig. Geometry and spatio-temporal evolution of a tiny seismic cluster. (a) Map view of the relocated events boosted by a ML2.2 event. The relocated hypocenters are shown as circles that are scaled to earthquake magnitude and colored to hypocentral depth. Blue beach-balls denote focal mechanisms determined by P-wave polarity data for events with ML greater than 1.3. Gray arrows show a possible Riedel shear structure. The inset shows a frequency distribution of the hypocenters as a function of the distance perpendicular to the fault-strike. (b, c) Depth sections of the relocated events perpendicular and parallel to the fault-strike, respectively. (d–f) Space–time diagram and an M–T diagram of the relocated events associated with the ML2.2 event, respectively.
Foreshock migration preceding the 2016 Mw 7.0 Kumamoto earthquake, Japan
We investigated the spatio-temporal evolution of an earthquake sequence following a series of large shallow intraplate earthquakes, including a Mw 6.2 foreshock and Mw 7.0 mainshock, in the Kumamoto area of Kyushu, SW Japan. To more precisely characterize the evolution of the earthquake sequences, we applied a matched filter technique to continuous waveform data, using template events obtained via a double-difference relocation algorithm. Migrations of seismicity fronts along the directions of fault strike and dip are clearly seen, starting immediately after the Mw 6.2 foreshock. These migrations are interpreted to result from aseismic slip triggered by the foreshock, propagating towards the nucleation point of the subsequent Mw 7.0 mainshock rupture. When combined with static stress changes induced by the Mw 6.2 foreshock, it is likely that stress transfer from both aseismic and seismic slip during the foreshock sequence loaded stress onto the mainshock rupture faults, bringing them closer to failure (Kato et al., 2016, Geophys. Res. Lett.).
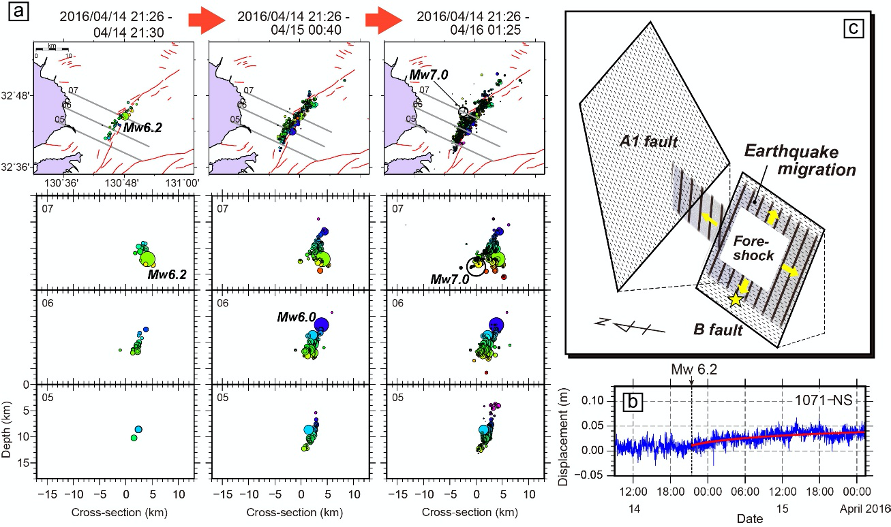
Fig. Spatio-temporal evolution of seismicity during the period between the foreshock and mainshock ruptures. (a) Plots of the cumulative distribution of epicentral locations over time, in map view and cross-section, are shown in the top and bottom panels, respectively. Events are colored according to hypocentral depth. (b) Time-series of displacement recorded at GNSS station 1071 (north–south component). The foreshock origin time is denoted by the dashed line. The logarithmic curve that best fits the transient deformation following the foreshock rupture is shown by the red line. (c) Perspective view of foreshock slip and aseismic slip propagation on the mainshock rupture planes.
Accelerated step-like unlocking of fault before ethe 2014 Iquique, Chile Mw 8.2 Earthquake
The earthquake nucleation process has been vigorously investigated based on geophysical observations, laboratory experiments, and theoretical studies; however, a general consensus has yet to be achieved. Here, we studied nucleation process for the 2014 Iquique, Chile Mw 8.2 megathrust earthquake located within the current North Chile seismic gap, by analyzing a long-term earthquake catalog constructed from a cross-correlation detector using continuous seismic data. Accelerations in seismicity, the amount of aseismic slip inferred from repeating earthquakes, and the background seismicity, accompanied by an increasing frequency of earthquake migrations, started around 270 days before the mainshock at locations up-dip of the largest coseismic slip patch. These signals indicate that repetitive sequences of fast and slow slip took place on the plate interface at a transition zone between fully locked and creeping portions. We interpret that these different sliding modes interacted with each other and promoted accelerated unlocking of the plate interface during the nucleation phase (Kato et al., 2016, Scientific Reports).
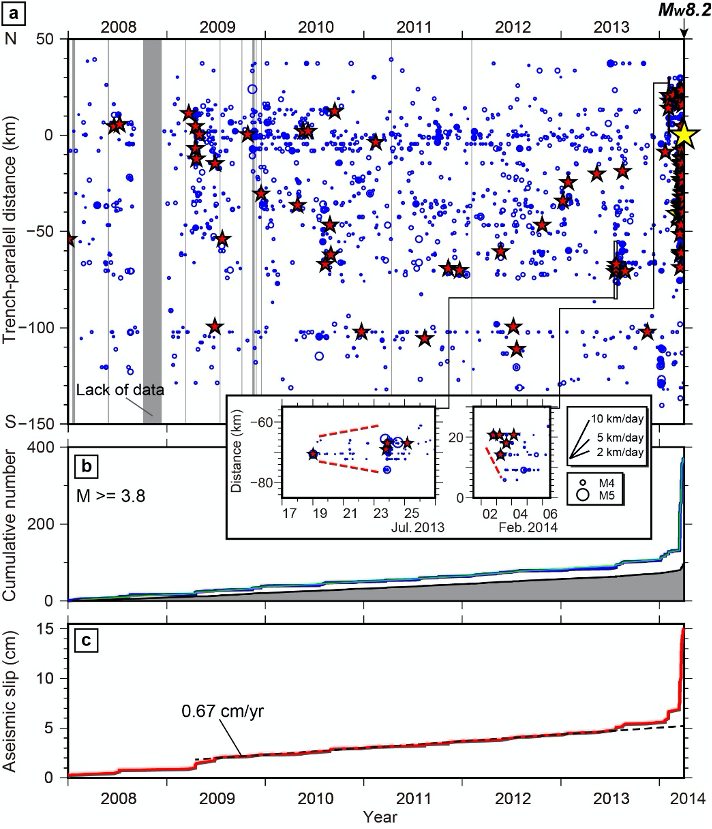
Fig. Decadal evolution of seismicity revealed by matched filter processing. (a) Space–time diagram of all detected events (blue circles) before the 2014 Iquique, Chile Mw 8.2 earthquake. Red stars indicate repeating earthquakes. Yellow star denotes the hypocenter of the mainshock. Periods of insufficient data are indicated by gray vertical bars. The insets show an expanded view of two seismic bursts in July 2013 and February 2014. Red dashed lines represent the approximate locations of the fronts of earthquake migrations. (b) Observed (blue thick line) and ETAS-modeled (green line) cumulative number of earthquakes with magnitude ≥ 3.8. Black line denotes temporal variation of cumulative number of background seismicity (grey shaded area). (c) Cumulative displacement of aseismic slip deduced from all groups of the repeating earthquakes.
Propagation of slow slip leading up to the 2011 Mw 9.0 Tohoku-Oki earthquake
Many large earthquakes are preceded by one or more foreshocks, but it is unclear how these foreshocks relate to the nucleation process of the mainshock. On the basis of an earthquake catalog created using a waveform correlation technique, we identified two distinct sequences of foreshocks migrating at rates of 2–10 km/day along the trench axis toward the epicenter of the 2011 Mw 9.0 Tohoku-Oki earthquake. The time history of quasi-static slip along the plate interface, based on small repeating earthquakes that were part of the migrating seismicity, suggest that two sequences involved slow slip transients propagating toward the initial rupture point. The second sequence, which involved large slip rates, may have caused substantial stress loading, prompting the unstable dynamic rupture of the mainshock (Kato et al., 2012, Science).
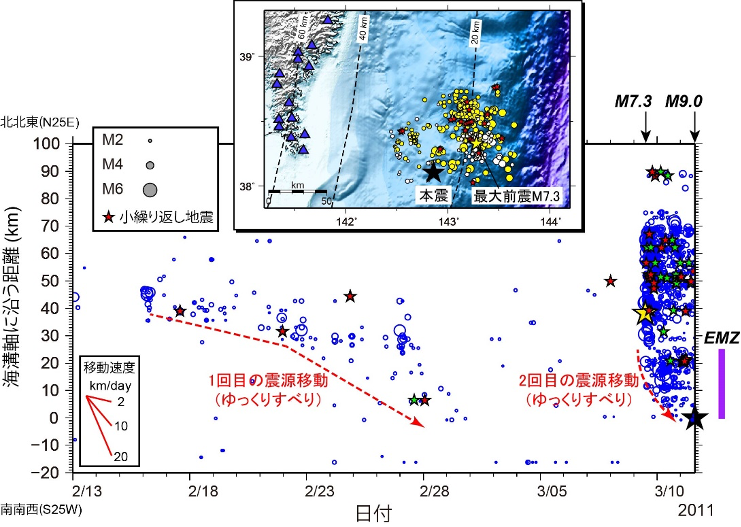
Fig. Earthquake migration toward the rupture initiation point of the mainshock (Kato et al., 2012, Science). Space-time diagram of all detected events between 13 February and the mainshock origin time, with earthquake origin locations indicated in terms of the distance along the trench axis (blue circles scaled to magnitude). Red dashed lines, approximate locations of the fronts of earthquake migration. Red stars, repeating earthquakes in the JMA catalog (23). Green stars, newly detected events that were found to resemble those repeating events. Black star, Mw 9.0 mainshock. Yellow star, Mw 7.3 largest foreshock. The inset shows time variations in seismicity rates inside and outside the EMZ following the Mw 7.3 largest foreshock. Blue broken curve, least squares fitting of the modified Ohmori law.